
GO Faster Go Further
Established since 2001
Secure Supplies Group
Electrical Polarization Process
VOLTROLYSIS
NOT! Electrolysis
(no electrolytes present)

Polarization in a Water Capacitor
A capacitor is a device that stores electrical energy in an electric field by separating charges on two conductive plates/ Tubes.
The Fermi Level Barrier
The Fermi level is a concept used in solid-state physics to describe the energy level at which el...
Ionization of the Water Molecule
In a tubular water-filled capacitor, water molecules are ionized due to the presence of an electr...
The Dielectric Constant of Water
The dielectric constant, also known as the relative permittivity, is a measure of a material's ab...
Hydrogen Bonds
Hydrogen bonds are a type of intermolecular force that occurs when a hydrogen atom, covalently bo...
Pulse Period Manipulation and Polarization
Electrical pulse period manipulation and polarization in a water capacitor is a fascinating area ...
Molecule & Gas Atom Ionization
- Raising H+ to 13.6 electronVolts
If you're looking to orient water molecules in an electric field, a relatively modest electric fi...
Water Molecule Bonds
1. The Water Molecule (H2O)
A water molecule consists of two hydrogen atoms and one oxygen atom. This molecule is not linear; instead, it forms a V-shape or a bent shape due to the presence of two lone pairs of electrons on the oxygen atom. The bond angle between the hydrogen-oxygen-hydrogen atoms is approximately 104.5 degrees.
Water is a polar molecule. This means that the molecule has a positive charge on one side (where the hydrogen atoms are located) and a negative charge on the other side (where the oxygen atom is). This occurs because oxygen is more electronegative than hydrogen, pulling the electrons closer and creating a partial negative charge on the oxygen and a partial positive charge on the hydrogens.
2. Types of Bonds in a Water Molecule
There are two types of bonds in a water molecule: covalent bonds and hydrogen bonds.
Covalent bonds: These are the bonds that hold the hydrogen atoms to the oxygen atom within a single water molecule. Each of the two hydrogen atoms shares a pair of electrons with the oxygen atom, forming a covalent bond.
Hydrogen bonds: These are the bonds between different water molecules. The partially positive hydrogen atom of one water molecule is attracted to the partially negative oxygen atom of another water molecule, forming a hydrogen bond. Hydrogen bonding is responsible for many of water's unique properties, such as its relatively high boiling point and its ability to dissolve many substances.
3. Water-Related Ions
Water can participate in reactions that produce ions. The two most common of these are the hydronium ion (H3O+) and the hydroxide ion (OH-).
Hydronium ion (H3O+): In the presence of an acid, a water molecule can gain a proton (H+) to become a hydronium ion. This is often simplified in equations as H2O + H+ -> H3O+.
Hydroxide ion (OH-): In the presence of a base, a water molecule can lose a proton to become a hydroxide ion. This can be represented as H2O -> H+ + OH-.
Water can also self-ionize, a process in which two water molecules produce a hydronium ion and a hydroxide ion: 2H2O -> H3O+ + OH-. This is a reversible reaction, and in pure water at room temperature, the concentrations of hydronium ions and hydroxide ions are both 1.0 x 10^-7 M, giving water a neutral pH of 7.
Alternative Bonds
Ionic bonds are formed when atoms exchange electrons. This usually happens between a metal and a non-metal. One atom (the metal) donates one or more electrons to the other atom (the non-metal). This creates ions: the metal becomes a positively charged cation, and the non-metal becomes a negatively charged anion. The attraction between these oppositely charged ions forms an ionic bond.
Metallic bonds
are found in metals. In these bonds, the metal atoms contribute their valence electrons to form a 'sea' of delocalized electrons. These free electrons move around the positively charged metal cations, holding the metal atoms together and contributing to the metal's electrical conductivity, malleability, and ductility.
Now, considering your scenario, where water is in a capacitor with 304L stainless steel (SS) electrodes and a non-standard molecule dissociation process is applied:I'm glad you found the information helpful. As for your new question, let's first understand the basic concepts of ionic and metallic bonds:
Ionic bonds
are formed when atoms exchange electrons. This usually happens between a metal and a non-metal. One atom (the metal) donates one or more electrons to the other atom (the non-metal). This creates ions: the metal becomes a positively charged cation, and the non-metal becomes a negatively charged anion. The attraction between these oppositely charged ions forms an ionic bond.
Metallic bonds are found in metals. In these bonds, the metal atoms contribute their valence electrons to form a 'sea' of delocalized electrons. These free electrons move around the positively charged metal cations, holding the metal atoms together and contributing to the metal's electrical conductivity, malleability, and ductility.
When a voltage is applied to the capacitor, the stainless steel electrodes can indeed inject electrons into the water. This can influence the water molecules and any ions or other compounds that may be dissolved in the water.
The addition of these extra electrons to the water can disrupt the balance of charges in the water molecules and can induce ionization, breaking the covalent bonds in the water molecule, causing it to split into hydrogen (H2) and oxygen (O2) gases, a process known as electrolysis.
The injected electrons from the stainless steel would be more likely to interact with the hydronium (H3O+) and hydroxide (OH-) ions present in water. These ions have a charge and are, therefore, more likely to interact with the excess or lack of electrons. In particular, the hydroxide ions (OH-) might attract the injected electrons, possibly leading to the formation of hydrogen gas (H2) and oxygen ions (O2-) which could then pick up protons from hydronium ions (H3O+) to form water again.
This process would not involve ionic or metallic bonding directly but instead involves a kind of redox (reduction-oxidation) reaction, where electrons are transferred from one species to another.
When a voltage is applied to the capacitor, the stainless steel electrodes can indeed inject electrons into the water. This can influence the water molecules and any ions or other compounds that may be dissolved in the water.
The addition of these extra electrons to the water can disrupt the balance of charges in the water molecules and can induce ionization, breaking the covalent bonds in the water molecule, causing it to split into hydrogen (H2) and oxygen (O2) gases, a process known as electrolysis.
The injected electrons from the stainless steel would be more likely to interact with the hydronium (H3O+) and hydroxide (OH-) ions present in water. These ions have a charge and are, therefore, more likely to interact with the excess or lack of electrons. In particular, the hydroxide ions (OH-) might attract the injected electrons, possibly leading to the formation of hydrogen gas (H2) and oxygen ions (O2-) which could then pick up protons from hydronium ions (H3O+) to form water again.
This process would not involve ionic or metallic bonding directly but instead involves a kind of redox (reduction-oxidation) reaction, where electrons are transferred from one species to another.
Polarization in a Water Capacitor
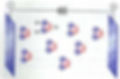
Note Always use tube cell snot flat plates as you wil l get 4.75 x times more gas from the distilled water no salts as plates not hold capacitance well.
A capacitor is a device that stores electrical energy in an electric field by separating charges on two conductive plates that are separated by a non-conductive material called a dielectric. In the case of a water-filled capacitor with two tubular plates, the dielectric is the water itself. This type of capacitor is called an electrolytic capacitor, and it has some unique properties due to the polarization of the water molecule.
The water molecule is a polar molecule, meaning that it has a positive charge on one end and a negative charge on the other. This polarization is due to the unequal distribution of electrons in the molecule, which causes the oxygen atom to have a slight negative charge and the hydrogen atoms to have a slight positive charge.
When a voltage is applied to the two tubular plates in the water-filled capacitor, the polar water molecules align themselves with the electric field, with the positive ends of the molecules facing the negative plate and the negative ends facing the positive plate. This causes a separation of charge in the water, with the positive ions accumulating near the negative plate and the negative ions near the positive plate.
The dielectric constant of a material is a measure of how easily it becomes polarized in an electric field. The dielectric constant of water is relatively high, which means that it is easily polarized by an applied electric field. This makes water a good dielectric material for use in electrolytic capacitors.
The 304L stainless tubing used in the water-filled capacitor is a good conductor of electricity, but it has a relatively low breakdown voltage.
This means that if the voltage across the capacitor exceeds a certain threshold, the tubing can break down and become damaged. To increase the voltage threshold of the capacitor, the tubing can be doped with Cr2O3, which is a ceramic material that has a high dielectric strength. When the tubing is doped with Cr2O3, it becomes a better insulator and can withstand higher voltages without breaking down.
In summary,
the electrical polarization of the water molecule in a water-filled capacitor with two tubular plates is an important factor in the capacitor's performance. The high dielectric constant of water allows for efficient energy storage, while the use of Cr2O3-doped 304L stainless tubing can increase the voltage threshold of the capacitor and prevent damage due to breakdown.
The Fermi Level Barrier

The Fermi level is a concept used in solid-state physics to describe the energy level at which electrons in a material have a 50% probability of being occupied. In the case of natural water and a 304L stainless steel electrode, the behavior of the Fermi level is important in understanding the electrochemical reactions that occur at the electrode-water interface.
Natural water, such as that found in lakes, rivers, and oceans, is a complex mixture of dissolved gases, ions, and organic compounds. These components can interact with the electrons in the water and affect the position of the Fermi level.
The Fermi level of natural water is generally close to the energy level of the water's highest occupied molecular orbital (HOMO), which is the energy level of the highest occupied electrons in the water molecule.
304L stainless steel is a commonly used material for electrodes in electrochemical applications due to its excellent corrosion resistance and durability.
The Fermi level of the metal can be affected by several factors, including the presence of impurities, defects, or surface treatments. In the absence of any external influences, the Fermi level of 304L stainless steel is typically located within the energy band gap of the material.
When a 304L stainless steel electrode is submerged in natural water, electrochemical reactions can occur at the electrode-water interface.
These reactions involve the transfer of electrons between the electrode and the water, which can cause changes in the position of the Fermi level. If the electrode is more electronegative than the water, electrons will flow from the water to the electrode, causing the Fermi level of the water to decrease and the Fermi level of the electrode to increase.
This can result in the formation of an electric double layer at the electrode-water interface, which can affect the behavior of ions and molecules in the water.

The behavior of the Fermi level of natural water and a 304L stainless steel electrode is important in many electrochemical applications, including corrosion prevention, electroplating, and water treatment.
Understanding the electrochemical reactions that occur at the electrode-water interface can help to optimize these processes and improve their efficiency and effectiveness.
The position of the Fermi level can be affected by several factors, including the composition of the water, the surface condition of the electrode, and the electrochemical reactions that occur at the electrode-water interface. By understanding these factors, researchers and engineers can develop new and improved methods for electrochemical applications.
See Stephen Meyer's Radio Interviews for his unique perspective on the birth of the Schottkey diode, the behavior of the P-metal junction and how natural water introduces a doping effect on the SS 304L capacitor plate.
"Don't ask, what the metal electrode introduces to the water. Ask, what the water introduces to the metal electrode." it becomes a Diode.
~ Stephen Meyer
The Schottky diode is a semiconductor device that has revolutionized electronics since its discovery in the early 20th century. It is named after the German physicist Walter H. Schottky who first observed the phenomenon of rectification in metal-semiconductor junctions in 1938. Schottky diodes are used in a wide range of electronic applications, from power conversion to radio frequency signal detection.
The basic principle behind the Schottky diode is the manipulation of the Fermi level of a semiconductor by the introduction of a metal layer. When a metal is deposited on a semiconductor material, it forms a junction that allows current to flow in only one direction. The metal-semiconductor junction behaves like a diode, allowing current to flow in only one direction, from the metal to the semiconductor.
The Schottky diode is different from a conventional p-n junction diode in that it has a metal-semiconductor junction, rather than a p-type and n-type semiconductor junction. The metal layer in the Schottky diode is typically made of a noble metal such as gold, platinum, or tungsten. The semiconductor material can be either p-type or n-type. The metal layer is deposited onto the semiconductor material through a process known as sputtering.
Schottky diodes have several advantages over p-n junction diodes. They have a lower forward voltage drop, faster switching speed, and lower reverse recovery time. These properties make them ideal for high-frequency applications such as radio frequency detection and power conversion.
Experiments with P-metal and N-metal junctions have shown that doping can create either a resistive-like connection or a diode-like connection.
Doping is the process of introducing impurities into a semiconductor material to alter its electrical properties. In p-type semiconductors, the doping introduces acceptor impurities, which create holes in the valence band. In n-type semiconductors, the doping introduces donor impurities, which create electrons in the conduction band.
By doping the semiconductor material, the Fermi level can be shifted, creating either a resistive-like connection or a diode-like connection. In a resistive-like connection, the Fermi level is near the middle of the bandgap, and the current flows in both directions. In a diode-like connection, the Fermi level is closer to the conduction or valence band, depending on the type of semiconductor material, and the current flows in only one direction.
The discovery of the Schottky diode and experiments with P-metal and N-metal junctions have greatly contributed to the development of modern electronics.
Schottky diodes have found wide applications in areas such as power conversion, radio frequency detection, and digital electronics.
The ability to manipulate the Fermi level of semiconductor materials through doping has enabled the development of advanced semiconductor devices such as transistors, integrated circuits, and light-emitting diodes.
Ionization of the Water Molecule
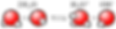
In a tubular water-filled capacitor, water molecules are ionized due to the presence of an electric field between the two tubular plates. This ionization process is important in understanding the behavior of the capacitor, as it can lead to dielectric breakdown and other issues.
When an electric field is applied to the water-filled capacitor, the water molecules become polarized, with the positive ends of the molecules orienting towards the negative plate and the negative ends orienting towards the positive plate.
As the electric field increases, the polarization becomes stronger, and some of the water molecules are ionized, releasing H+ and OH- ions into the water.
Over-ionization can occur if the electric field becomes too strong, leading to the breakdown of the dielectric and the formation of a conducting path between the two plates.
This can cause a sudden discharge of the stored energy in the capacitor, leading to damage or failure of the system.
Dielectric breakdown can also occur if the water contains impurities or dissolved ions that can enhance the conductivity of the water and decrease the dielectric strength.

The ionization process in the water-filled capacitor leads to the formation of H+ and OH- ions in the water.
The H+ ions are positively charged and are attracted towards the negative plate, while the OH- ions are negatively charged and are attracted towards the positive plate.
This movement of ions in the water creates an electric current, which can affect the behavior of the capacitor and lead to issues such as self-discharge and leakage current.
The creation of H+ and OH- ions in the water-filled capacitor can also have other effects, such as affecting the pH of the water.
As H+ ions are released into the water, the pH of the water becomes more acidic, while the release of OH- ions can make the water more basic. The pH of the water can affect the behavior of the capacitor, as changes in pH can alter the conductivity and dielectric properties of the water.
In conclusion, the ionization of water molecules in a tubular water-filled capacitor is an important process that can affect the behavior of the capacitor.
Over-ionization can lead to dielectric breakdown, while the creation of H+ and OH- ions can affect the pH and conductivity of the water. Understanding these processes is important in designing and operating water-filled capacitors for various applications.
The Dielectric Constant of Water
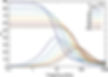
I'm a paragraph. Click here to add your own text and edit me. It's easy.
The dielectric constant, also known as the relative permittivity, is a measure of a material's ability to store electrical energy in an electric field. In the case of water, the dielectric constant is relatively high, making it an effective dielectric material for use in electrical circuits.
When water is used as a dielectric material in an electrical circuit, it behaves as a resistor, limiting the flow of electrical current through the circuit. The resistance of the water depends on several factors, including the concentration of ions and impurities in the water, the temperature, and the applied electric field.
The resistance of water as a dielectric material can be modeled using Ohm's Law, which states that the current flowing through a resistor is directly proportional to the voltage across the resistor and inversely proportional to the resistance of the resistor. In the case of water, the resistance can be affected by the dielectric constant, which determines the amount of electrical energy that can be stored in the water.
The dielectric constant of water is influenced by the presence of ions and molecules in the water, as well as the temperature and pressure of the water. At room temperature and standard pressure, the dielectric constant of water is approximately 80, making it an effective dielectric material for use in electrical circuits.
The dielectric constant of water also influences the capacitance of the circuit, which is a measure of the amount of electrical charge that can be stored in the circuit for a given voltage.
The capacitance of a circuit containing water as a dielectric material can be calculated using the equation C = εA/d, where C is the capacitance, ε is the dielectric constant of water, A is the area of the plates in the capacitor, and d is the distance between the plates.
In addition to its use as a dielectric material, water can also act as a capacitor itself, storing electrical charge in the form of ions and molecules in the water. This can lead to self-discharge and leakage currents in the circuit, which can affect the performance of the circuit over time.

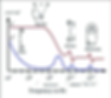
The relative permittivity,
also known as the dielectric constant, of water in a capacitor is an important property that affects the behavior of the capacitor.
The dielectric constant of water
varies with frequency and temperature, and different frequency ranges can have different effects on the various types of polarizability in water.
Ionic polarizability in water occurs when ions in the water are attracted to the opposite charge on the plates of the capacitor, causing
the water to become polarized. At low frequencies, ionic polarizability dominates, and the dielectric constant of water increases.
This can cause the capacitance of the capacitor to increase as well, allowing it to store more charge at low frequencies.
Atomic and vibrational
polarizability in water occurs when the electric field of the capacitor causes the atoms and molecules in the water to vibrate, leading to polarization of the water molecules.
This type of polarizability is most effective at intermediate frequencies, typically between 1 MHz and 1 GHz. At these frequencies, the dielectric constant of water is high, and the water can store a significant amount of electrical charge.
Electric polarizability
in water occurs when the water molecules become aligned in response to the electric field of the capacitor. This type of polarizability is most effective at high frequencies, typically above 10 GHz. At these frequencies, the water molecules become polarized quickly, allowing the capacitor to store charge rapidly.
Dipolar polarizability
in water occurs when the water molecules themselves have an inherent dipole moment, causing them to become polarized in response to the electric field of the capacitor. This type of polarizability is most effective at frequencies below 1 MHz, where the dielectric constant of water is low.
In conclusion, the dielectric constant of water is an important property that influences its behavior as a resistor in an electrical circuit. Understanding the resistance and capacitance of water in electrical circuits is important in designing and optimizing the performance of electrical systems that utilize water as a dielectric material.
The relative permittivity of water in a capacitor depends on the frequency and temperature of the system. Different types of polarizability in water, such as ionic, atomic/vibrational, electric, and dipolar, are affected differently by different frequency ranges. Understanding these effects is important in designing and optimizing the performance of electrical systems that utilize water as a dielectric material.
Hydrogen Bonds
Hydrogen bonds are a type of intermolecular force that occurs when a hydrogen atom, covalently bonded to an electronegative atom, such as oxygen or nitrogen, interacts with a neighboring electronegative atom in another molecule. Hydrogen bonds are responsible for many important properties of substances, such as the high boiling point of water and the stability of protein and DNA structures.

In Voltrolysis
we use no NaCl or electrolytes
only distilled water
To understand how hydrogen bonds form, it is important to first understand the basic structure of a water molecule. A water molecule consists of two hydrogen atoms covalently bonded to a single oxygen atom. Due to the electronegativity of the oxygen atom, the electrons in the covalent bonds are more attracted to the oxygen atom, creating a partial negative charge on the oxygen and a partial positive charge on the hydrogen atoms.
When two water molecules come close together, the partial positive charge of one hydrogen atom is attracted to the partial negative charge of the oxygen atom in the other molecule, forming a hydrogen bond. This interaction is not as strong as a covalent bond, but it is strong enough to cause water molecules to stick together, which is why water has a high surface tension and can form droplets.
Hydrogen bonds are also responsible for the unique properties of water, such as its high boiling point and heat capacity.
When heat is added to water, the energy is used to break the hydrogen bonds between water molecules, rather than increasing the kinetic energy of the molecules. This means that water can absorb a lot of heat energy without changing temperature, making it an effective coolant.
Microwaves, on the other hand, heat water through a different mechanism. Microwaves are a form of electromagnetic radiation that can be absorbed by water molecules, causing them to vibrate rapidly and generate heat. This is why microwaves are effective at heating water-based substances, such as food or beverages.
In summary, hydrogen bonds are a type of intermolecular force that occurs when a hydrogen atom interacts with an electronegative atom in another molecule.
They are responsible for many important properties of substances, such as the high boiling point of water and the stability of protein and DNA structures. Microwaves, on the other hand, heat water through the absorption of electromagnetic radiation, causing water molecules to vibrate and generate heat.
Pulse Period Manipulation and Polarization
Electrical pulse period manipulation and polarization in a water capacitor is a fascinating area of study that has the potential to revolutionize the field of energy storage. In this article, we will explore the concepts of pulse period manipulation and polarization in water capacitors, their significance, and the current state of research in this field.
A capacitor is a device that stores electric charge. It consists of two conducting plates separated by an insulating material, also known as a dielectric. The capacitance of a capacitor depends on the surface area of the plates, the distance between them, and the properties of the dielectric. A water capacitor is a type of capacitor where the dielectric is water.

One of the interesting properties of water is that it can be polarized by an electric field. This means that when an electric field is applied to water, the water molecules align themselves with the field, creating a dipole moment. The degree of polarization depends on the strength of the electric field and the properties of the water.
Pulse period manipulation is a technique where electrical pulses are applied to a water capacitor at specific time intervals. The pulse period refers to the time interval between the application of successive pulses. By manipulating the pulse period, researchers can control the polarization of the water in the capacitor.
Recent research has shown that pulse period manipulation can significantly increase the energy density of water capacitors. Energy density is a measure of the amount of energy stored per unit volume or mass. By controlling the polarization of the water, researchers can increase the energy density of the capacitor, making it a more efficient energy storage device.

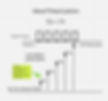
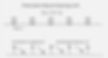
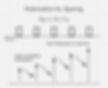

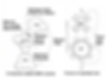
In addition to pulse period manipulation, researchers are also studying the effects of electrode materials on the polarization of water capacitors. The choice of electrode material can have a significant impact on the performance of the capacitor.
For example, research has shown that using carbon-based electrodes can enhance the polarization of water, leading to higher energy densities.
Water capacitors have several advantages over traditional energy storage devices, such as batteries. They are non-toxic, non-flammable, and have a longer lifespan. In addition, they can store large amounts of energy and can be charged and discharged quickly.

Molecule & Gas Atom Ionization - Raising H+ to 13.6 electronVolts
If you're looking to orient water molecules in an electric field, a relatively modest electric field can achieve this because water is a polar molecule. To align a water molecule, an electric field on the order of 1 kV/cm is often more than enough. Given a 1/8" gap (approximately 0.318 cm), this would equate to a voltage of approximately 318 volts.
In its neutral state, a hydrogen atom is not electropositive. The hydrogen atom consists of one proton and one electron, and it is electrically neutral because the positive charge of the proton balances the negative charge of the electron. However, hydrogen can become a positive ion (a proton) if it loses its electron, such as through ionization.
If you're dealing with ionized hydrogen (protons), these would indeed be repelled by a positive electric field and attracted to a negative one. If the hydrogen atoms are not ionized, they would not be significantly affected by an electric field because they are overall electrically neutral.
If you're trying to manipulate neutral hydrogen atoms with an electric field, you may need to ionize them first. Ionization typically requires a significant amount of energy and can be achieved in various ways, such as with a high voltage, intense light, or high temperatures.
The process of converting a neutral hydrogen atom into a positively charged ion (a proton, H+) is called ionization. For hydrogen, this involves removing the single electron from the atom. There are several methods that can be used to achieve this:
-
Electrical Discharge: Passing a high-voltage electric current through the gas can strip electrons from their parent atoms, creating ions. This is the principle behind techniques like glow discharge and arc discharge.
-
Electron Impact: High-energy electrons can knock electrons off atoms to create ions. This often happens in devices like mass spectrometers.
-
Photoionization: High-energy photons (such as ultraviolet light or X-rays) can also ionize atoms by knocking off electrons.
-
Thermal Ionization: At very high temperatures, the kinetic energy of the atoms can be high enough to cause ionization. This is what happens in the sun and other stars.
-
Chemical Ionization: Certain chemical reactions can result in ionization. For example, some strong acids can ionize hydrogen.
Sure, let's delve deeper into these three methods:
-
Electrical Discharge Ionization: This is the process of ionizing a gas by applying a high voltage between two electrodes. When the voltage is sufficiently high, it can accelerate free electrons, which then collide with neutral gas atoms. If the kinetic energy of the electron is high enough, it can knock an electron off the atom, creating an ion. This process can create a plasma, a gas of ions and free electrons.
Glow discharge and arc discharge are two forms of electrical discharge. Glow discharge operates at lower pressures and voltages, while arc discharge operates at higher pressures and voltages.
In your context, you could potentially use an electrical discharge to ionize your hydrogen gas. You would need to carefully contain and control the discharge to ensure safety, as the process can produce heat and UV light.
-
Electron Impact Ionization (also known as Collision Ionization): This method is similar to electrical discharge but is usually discussed in the context of a beam of high-energy electrons colliding with a gas. When a high-energy electron collides with a neutral atom, it can knock off one of the atom's electrons, creating an ion.
This method is often used in instruments like mass spectrometers to ionize samples. It's typically used in vacuum conditions, as the mean free path of the electrons needs to be long enough for them to gain sufficient energy before they collide with the atoms.
-
Photoionization: This is the process of ionizing an atom using photons, which are particles of light. When a photon with enough energy collides with an atom, it can knock an electron off the atom, creating an ion. The minimum energy required to ionize the atom is called the ionization energy.
For hydrogen, the ionization energy is 13.6 electron volts (eV), which corresponds to ultraviolet light with a wavelength of about 91.2 nm. So you would need light of this energy or higher to photoionize hydrogen. Photoionization can be a very clean method of ionization, as it doesn't introduce any new particles into the system. However, producing light of the required energy can be challenging. UV lasers can do this, but they tend to be expensive and require careful handling due to the hazards of UV light.
Again, all of these methods involve significant energy and can be hazardous if not handled correctly. Always follow appropriate safety procedures, and consider consulting with an expert if you're unsure.
The electronvolt (eV) is a unit of energy that is often used in the context of atoms and subatomic particles, like electrons. One electronvolt is defined as the amount of kinetic energy gained (or lost) by a single electron accelerating from rest through an electric potential difference of one volt.
So, when we say that the ionization energy of hydrogen is about 13.6 eV, it means that it takes 13.6 eV of energy to remove an electron from a hydrogen atom (in its ground state).
This energy isn't directly related to the input voltage of a circuit or device. The input voltage can create an electric field, and this field can accelerate charged particles. If a charged particle (like an electron) accelerates through a voltage difference of one volt, it will gain (or lose) an energy of one electronvolt.
But to use voltage to ionize an atom, you need a situation where free electrons can be accelerated by the electric field to gain enough kinetic energy.
This can happen in an electrical discharge, for example, where a high voltage can ionize a gas. The voltage needed to achieve this can be much higher than the ionization energy of the atoms (in eV), due to inefficiencies and losses in the process. The specific voltage needed can depend on many factors, such as the pressure and composition of the gas, the distance between the electrodes, and the design of the device.
As always, dealing with high voltages and ionization processes can involve significant hazards, and it's important to follow all relevant safety procedures and guidelines.
The water capacitor is 2 - 3" tubes, 1/2" inner and 3/4" outer SS304L. the gap is approx 1/8". what do the electric field voltage requirements look like for this?
The electric field strength (E) in a capacitor is given by the formula E = V/d, where V is the voltage across the capacitor and d is the distance between the plates (or in this case, tubes). The electric field is usually measured in volts per meter (V/m).
The 1/8" gap between your tubes is about 0.003175 meters. If you were to apply a voltage of 1 volt across the tubes, the electric field strength would be E = 1V / 0.003175m = 315 V/m.
If you're looking to ionize hydrogen, as discussed earlier, you'd need an electric field that's strong enough to give electrons enough energy to escape their atoms. This would likely require a field on the order of kilovolts per millimeter or higher. For a 1/8" (about 3.175mm) gap, this could mean a voltage of several kilovolts.
However, creating such a strong electric field in a gas at atmospheric pressure would likely lead to electrical breakdown and a spark or arc, rather than ionization of individual atoms. To achieve controlled ionization, you would typically need a vacuum or very low pressure, so the mean free path of the electrons is long enough for them to gain sufficient energy before they collide with another atom.
If you're simply looking to align water molecules with an electric field, you would not need such a high field strength. A field of a few kV/m (equivalent to a few volts across the tubes) could be sufficient.
In any case, working with high voltages can be dangerous, and it's important to take all necessary safety precautions. Consider consulting with an expert or professional if you're unsure.
what field strength is needed to apply the 13.6eV
In order to understand how much field strength is required to apply the 13.6 electronvolt (eV) needed to ionize a hydrogen atom, we need to take into account the concept of work done.
The work done (W) on a particle by an electric field as it moves through a distance (d) is given by W = qEd, where q is the charge of the particle and E is the electric field strength. If we want to give an electron an energy of 13.6 eV, we can set W equal to this value.
The charge of an electron (q) is about 1.6 x 10^-19 Coulombs. So, if we want to give an electron an energy of 13.6 eV (which is about 2.18 x 10^-18 Joules), we can rearrange the equation to solve for E:
E = W / (q*d) = (2.18 x 10^-18 J) / [(1.6 x 10^-19 C) * d]
For a 1/8" (about 0.003175 m) gap, this gives:
E = (2.18 x 10^-18 J) / [(1.6 x 10^-19 C) * 0.003175 m] ≈ 4.3 x 10^7 V/m
This means that you would need an electric field of about 43 million volts per meter to give an electron an energy of 13.6 eV over a distance of 1/8". That corresponds to a voltage of approximately 136.5 kV across the 1/8" gap.
However, these calculations are a simplification, and they assume that the electron is free to move across the entire gap without any collisions. In reality, the mean free path of an electron (the average distance it can travel before colliding with an atom) in air at atmospheric pressure is much less than 1/8", and so the electron would not be able to gain this much energy before it collided with an atom.
Therefore, to achieve ionization in a controlled manner, you would typically need a vacuum or very low pressure, and the voltage required would depend on the specific conditions.
As always, working with high voltages can be dangerous, and it's important to follow all necessary safety precautions. Consult with an expert or professional if you're unsure.
ok how about a 1/16" gap
With a reduced gap distance of 1/16" or approximately 0.0015875 meters, we use the same formula for the electric field:
E = W / (q*d)
To calculate the required voltage (V), we remember that electric field strength (E) is voltage (V) divided by distance (d). So we can rearrange the formula to find V = E*d:
V = E*d = W / q
Using the numbers we established before (W = 2.18 x 10^-18 J and q = 1.6 x 10^-19 C), we find:
V = (2.18 x 10^-18 J) / (1.6 x 10^-19 C) ≈ 13.625 kV
So the voltage required across the 1/16" gap to provide an electron with 13.6 eV of energy would be approximately 13.625 kV.
Again, in reality, achieving ionization under atmospheric pressure conditions would likely require higher voltages due to factors like electron collision and energy loss. Always take proper precautions when dealing with high voltage systems.
Te result is a magnitude of 10
1/16" = 13.6kV = 13.6eV
1/8" = 136.5kV = 13.6eV